Interplanetary Rock Star
Ken Williford, C’98, leads NASA’s
search for signs of life in the
ancient geology of Mars.
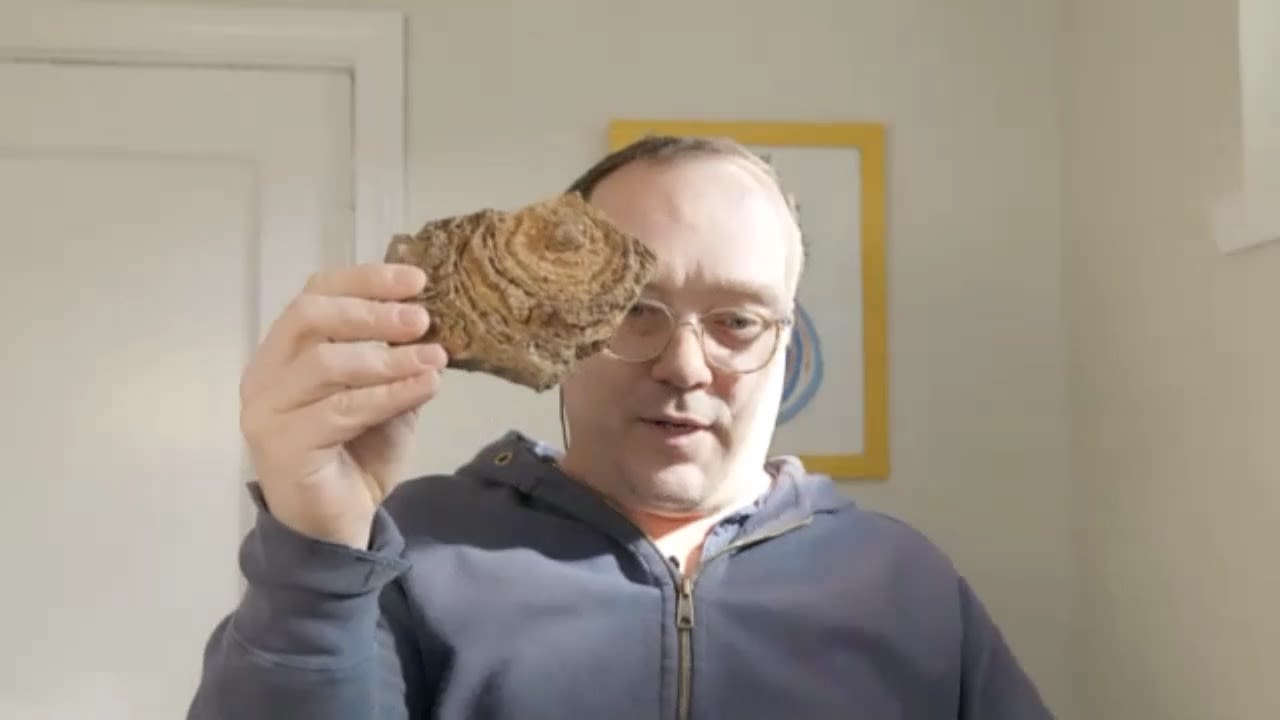
Ken Williford, C’98, remembers an essay prompt he faced as a high-school senior completing Sewanee’s application for the Wilkins Scholarship in 1994. It had to do with order and pattern in the universe, and it intrigued him because it was a topic he’d been fascinated by for most of his life. Is the universe governed by discernible laws or ruled by chaos? Can we find patterns that point to organizing principles? From all the inorganic matter that composes the universe, how did life on Earth arise?
Williford dates his interest in understanding the complexity and origins of the universe and living things to a trip his family took to the Philippines when he was just six years old. It was there, while he was snorkeling above a reef and gazing down on its wild profusion of life—the array of technicolor corals, the abundance and variety of fish—that a seed was planted. “I don’t know,” he says. “I just had this sense that I wanted to understand the life around me.”
His quest for understanding would lead Williford to Sewanee, to a Ph.D. in earth and space sciences at the University of Washington, and, ultimately, to Mars (or about as close as any of us is going to get to the red planet anytime soon). The former Sewanee natural resources major now serves as deputy project scientist for NASA’s Mars 2020 mission and is the director of the Astrobiogeochemistry Laboratory at NASA’s Jet Propulsion Laboratory (JPL). As one of the top scientists working on one of the most sophisticated attempts in human history to find signs of life beyond Earth, Williford is doing exactly what he’s done since he was a curious six-year-old in mask and fins: Asking the big questions and getting answers.
As a senior at a tiny independent high school in Dayton, Ohio, Williford was attracted to Sewanee’s small size and by a college-search guidebook that claimed, in his recollection, that the Domain was a place “where fuzzy-sweatered Bohemians kick Hacky Sacks around.” After reading that, all it took was a campus visit, and Williford was sold. “After driving through the gates, I just immediately knew, 100%, beyond the shadow of a doubt, this is where I want to be. It was the only place I applied, early admission.”
Though science was his first academic love, Williford came to Sewanee planning to major in English to pursue a dream of becoming a writer. But during his sophomore year, he took Geology 101 with Professor Martin Knoll and it became clear that studying rocks would open avenues to answering some of his questions about the nature of life itself. The obvious academic path for someone seeking insights into the living world would be biology, not geology, but to Williford, biology offered less expansive vistas than geology. “The way that I’m inspired to understand life is not the way biology tends to be taught, which is ‘Here are all the parts and pieces of life and how they fit together,’” he says. “I want to know what life is in its fundamental essence. With geology, it was about the whole system of the planet and how all the aspects worked together, life being a key part of that, and how that all fed back into itself.”
Knoll became his advisor, and Williford relished classes with Knoll and Bran Potter, where field labs were just a short hike or van ride away. He soaked up knowledge of soft-rock sedimentary geology on the Cumberland Plateau, which would become crucial to his later understanding of sedimentary geology on Mars. Another class he found particularly important to what would become his life’s work was a class on soils with Forestry Professor Scott Torreano that provided an important understanding of the interface between geology and biology.
“With geology, it was about the whole system of the planet and how all the aspects worked together, life being a key part of that, and how that all fed back into itself.”
After graduating from Sewanee and earning a master’s degree in geology from the University of Washington, Williford took a detour that included a stint teaching middle school science on St. Croix in the U.S. Virgin Islands. With a desire to start a record label, he enrolled in a master’s program for audio engineering at Middle Tennessee State University. Then, deciding he wanted to make movies, he entered a filmmaking program at the Savannah College of Art and Design before having another epiphany at the crossroads of art and science, not unlike the one he had in Sewanee years earlier. “I had this kind of existential science-vs.-art moment,” Willford says. “Which direction should I go? It all collapsed down to ‘I need to go back to grad school and focus on science.’” He returned to the University of Washington, where he earned a Ph.D. in earth and space sciences.
Before the Mars 2020 mission had been announced, Williford joined JPL in 2012 to set up an organic chemistry laboratory that would one day examine rock samples brought back to Earth. “Mars sample return” is the NASA shorthand for this prospect, and it’s been a major focus of the space agency for years, but one with a moving target date. “There’s been this joke forever that we’re always 10 years away from Mars sample return,” Williford says. “Now we’re actually 10 years away from Mars sample return.”
NASA came calling when it learned that Williford was doing research that held the promise of fundamentally changing the way very old rocks could be analyzed to search for signs of life. Normally, a rock sample would be ground up so that its bulk composition could be studied. But during a postdoctoral fellowship at the University of Wisconsin, Williford was experimenting with new analytical methods for measuring the composition of organic matter in rocks by using a fine-scale beam. Williford’s methods and NASA’s needs dovetailed. Because any samples brought back from Mars would necessarily be small, nondestructive analysis that worked on tiny bits of rock would be needed.
Once every 10 years, the National Academy of Sciences convenes a group of top scientists to discuss how NASA should prioritize its planetary science funding over the next decade. In 2012, the results of the decadal survey were announced, and at the very top of the list of priorities was “significant progress toward Mars sample return.” Around the same time, Williford was starting his job at JPL, and when he arrived, one of the first three emails he found in his inbox was the announcement that NASA would begin planning for a future mission to be called “Mars 2020.” For an astrobiogeochemist with a dream of advancing humanity’s knowledge about the fundamental essence of life, Williford was in the right place at the right time.
Because one of the primary goals of Mars 2020 is right in Williford’s professional wheelhouse—to search for signs of ancient life—he was integrally involved in the complex process to choose the landing site for the Perseverance rover. The team ultimately chose Jezero Crater, one of the few places on the planet that was clearly once the site of a crater lake. What makes it clear that water once stood here is the presence of an ancient river channel that flowed into the lake, another river channel that flowed out, and a delta. Deltas form when flowing water meets a standing body of water. And the site of an ancient standing body of water is likely to offer the best chance for finding signs of past life.
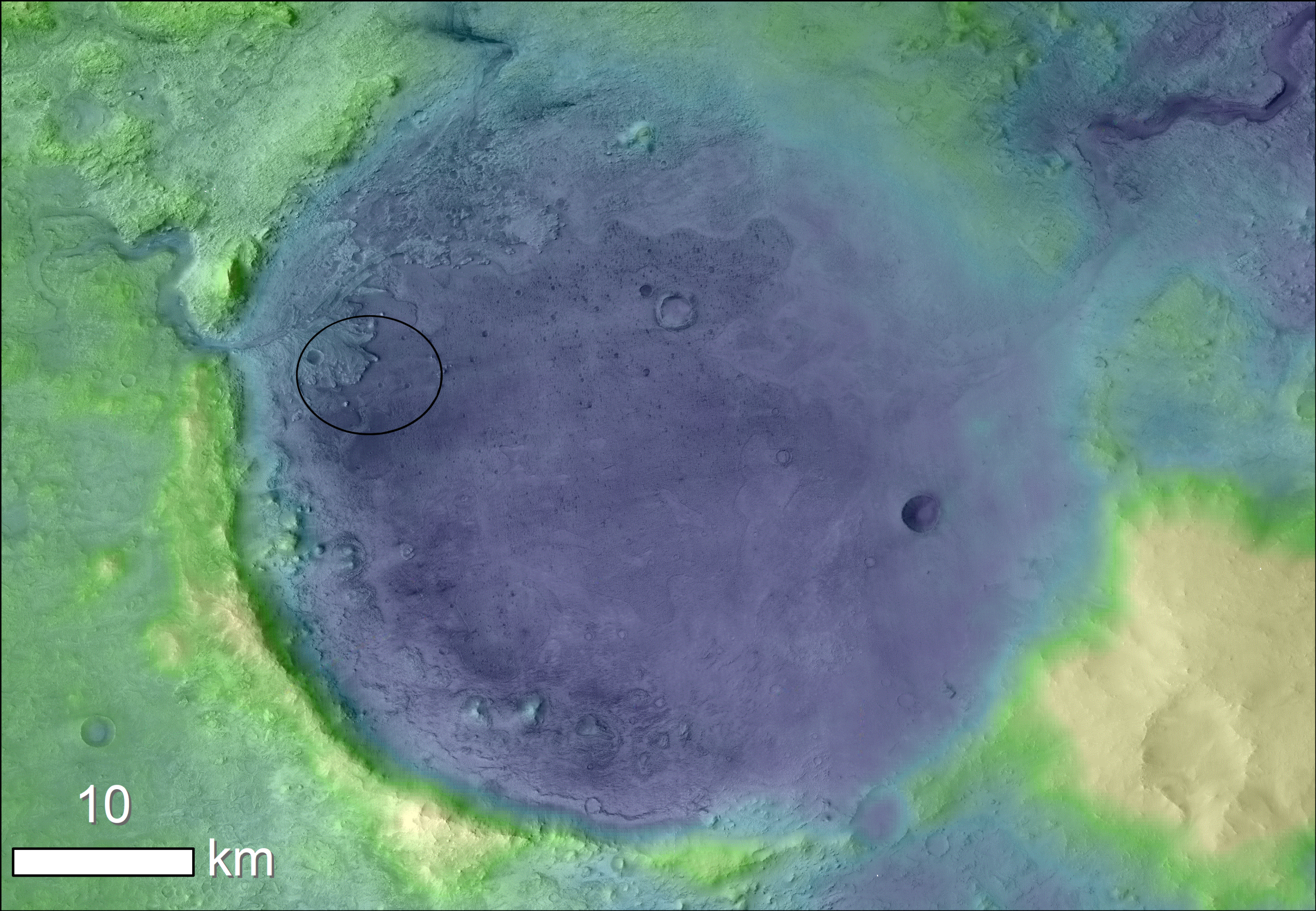
Lighter colors represent higher elevation in this image of Jezero Crater on Mars, the landing site for NASA's Mars 2020 mission. The black oval indicates the landing ellipse, where the rover touched down. The color added to this image helps the crater rim stand out clearly. The Mars 2020 teams hopes this shoreline might have preserved fossilized microbial life, if any ever formed on Mars. Image courtesy of NASA.
Lighter colors represent higher elevation in this image of Jezero Crater on Mars, the landing site for NASA's Mars 2020 mission. The black oval indicates the landing ellipse, where the rover touched down. The color added to this image helps the crater rim stand out clearly. The Mars 2020 teams hopes this shoreline might have preserved fossilized microbial life, if any ever formed on Mars. Image courtesy of NASA.
“Almost everything we know about the history of life on Earth comes from shallow-water environments,” Williford says. “There are a lot of things that live in shallow water, but perhaps more importantly, shallow-water environments are the best environments on Earth to preserve signs of ancient life. Almost our entire fossil record comes from shallow-water environments.”
Of course, Williford and the Mars 2020 team aren’t looking for dinosaur bones. Any evidence of life they might find will likely be signs of past microbial life. On Earth, the record of life stretches back nearly 4 billion years. But in the fossil record between 4 billion years ago and about half a billion years ago, the most complex life evident is all microbial. “The short version of what we’re looking for is lifelike shapes and lifelike compositions, especially when they occur together,” Williford says. “Lifelike elements, lifelike minerals, and lifelike organic molecules that are arranged in shapes, or morphologies, or textures that indicate biology.”
One example from Earth of the kind of microbial fossil Williford hopes to find on Mars is a stromatolite, and when we talked via Zoom recently, he had one on hand for a quick show and tell. The 2.7-billion-year-old domed fossil from Western Australia was formed when billions of microscopic bacteria got together in community to form wrinkly layers—or mats—at the edge of an ancient lake. The microbes exude a substance that traps sediment particles, and when minerals precipitate out of the lake water and the whole thing is buried, a fossil can form. Williford is clearly excited by this artifact from a distant era, while also admitting that “it’s basically a fossil version of the green goo that you might find at the edge of Lake Cheston.”
Perseverance is exploring Mars with a suite of sophisticated instruments that will aid in the search for signs of past microbial life, including PIXL (Planetary Instrument for X-ray Lithochemistry) and SHERLOC (Scanning Habitable Environments with Raman and Luminescence for Organics and Chemicals). PIXL and SHERLOC’s tools include X-ray and laser spectrometers that can identify chemical elements at a microscopic scale, and cameras for incredibly detailed photos of rocks and soil. But much of the most promising work will be done in labs like the one Williford has worked for years to set up at JPL to prepare for sample return, using tools that are far too large and heavy to send to Mars with the rover.
To get those precious samples of rock, Perseverance is equipped with a coring drill and 43 titanium sampling tubes. When the scientists find a rock they want to sample, a robotic arm on the front of the rover grabs a tube out of storage in the belly of the rover and puts it inside a drill bit. The rock is drilled, the sample is captured in the tube, and then the tube is sealed and returned to storage. Once Perseverance has collected 10 or so samples, they’ll be dropped in a “cache” on the ground, waiting to be picked up and returned to Earth. The rover will then move on to collect more samples and create more caches. In total, if everything goes as planned, about one pound of Mars rock will make its way back to Earth for further study in labs like Williford’s. The next opportunity to retrieve the samples will come in 2027 or 2028. Until then, the samples will sit in their tubes on the surface of Mars.
As Perseverance begins the search for promising rock samples on Mars, Williford’s team is conducting tests in a vacuum chamber at JPL. The process, known as QMDT (qualification model dirty testing), uses Earth rocks that might have properties similar to Mars rocks to test the rover’s drill. Because the forces that created Jezero Crater are similar to the forces that created the sedimentary geology of the South Cumberland Plateau—another ancient shallow-water environment—Williford knew that one kind of rock the drill needed to be tested on is a conglomerate. Conglomerates are made up of rounded rocks, “clasts,” that are suspended in a matrix of sand-sized particles and bound by a calcite or quartz cement. These rocks might pose a special challenge to Perseverance’s drill because of the differing material properties found in a single piece rock, especially when the coring drill meets a clast that’s about the same diameter as the inner diameter of the drill bit. When it came time to procure a sample of conglomerate to test, Williford knew just who to call.
He got in touch with his old Sewanee academic advisor, Martin Knoll, who was more than happy to help find a good-sized chunk of Sewanee conglomerate to ship to JPL. Knoll enlisted Assistant Professor of Geology Lily Thompson, who specializes in planetary geology and happened to be teaching a section of her Geology of Our Solar System course. Knoll, Thompson, and Thompson’s Solar System students trekked out on the Domain to locate a suitable candidate for drilling. They found one—a detached piece of conglomerate about a foot-and-a-half long, a foot wide, and 10 inches deep—near Piney Point. Knoll put the rock in a cooler, “duct-taped the hell out of it,” and took it to Mr. Postman in Winchester to send to Williford. “It’s probably the most expensive package I’ve ever shipped,” Knoll says.
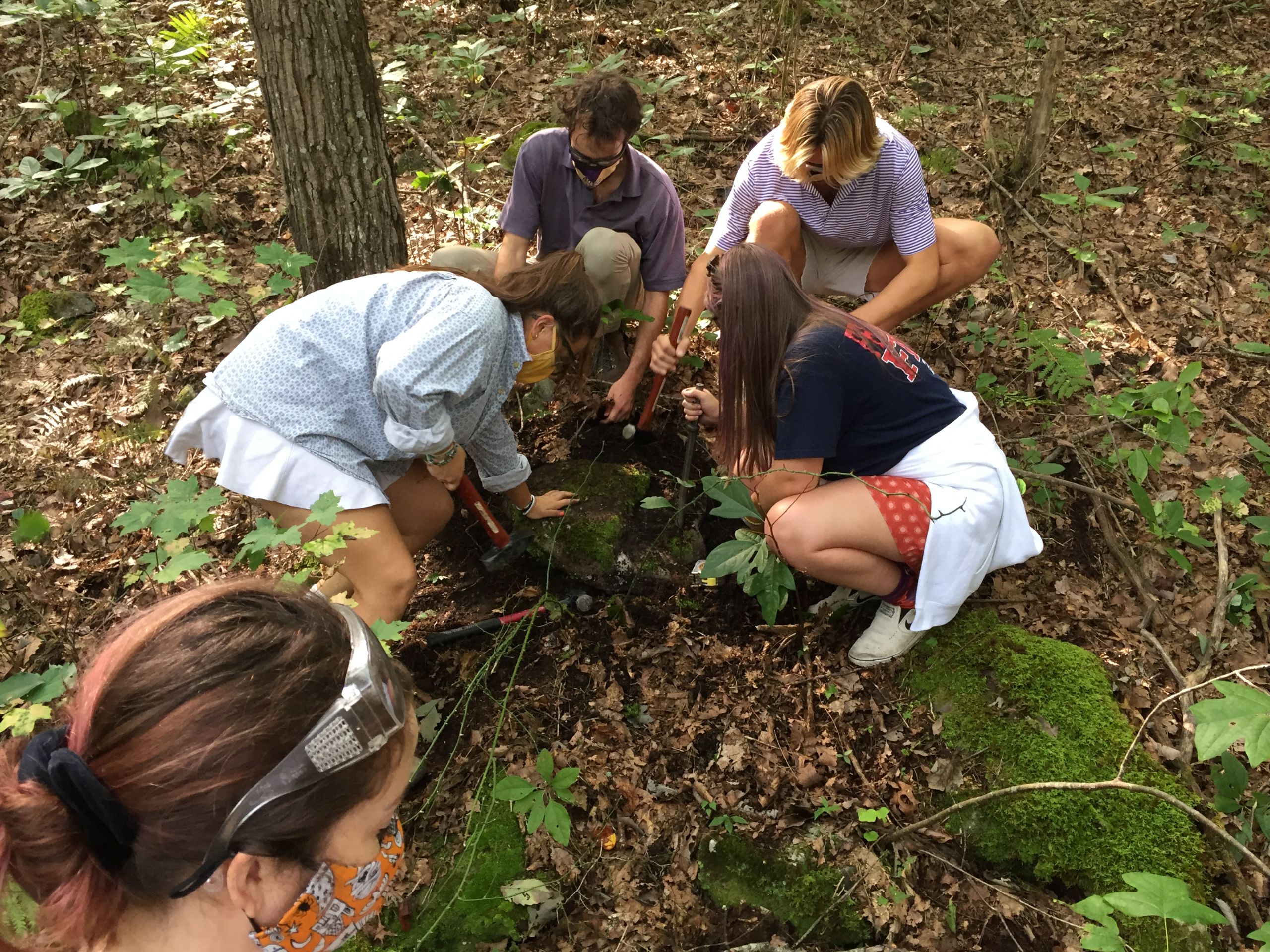
Lily Thompson's Geology of Our Solar System students hunt for the perfect piece of Sewanee conglomerate to send to JPL for testing. Photo courtesy of Martin Knoll.
Lily Thompson's Geology of Our Solar System students hunt for the perfect piece of Sewanee conglomerate to send to JPL for testing. Photo courtesy of Martin Knoll.
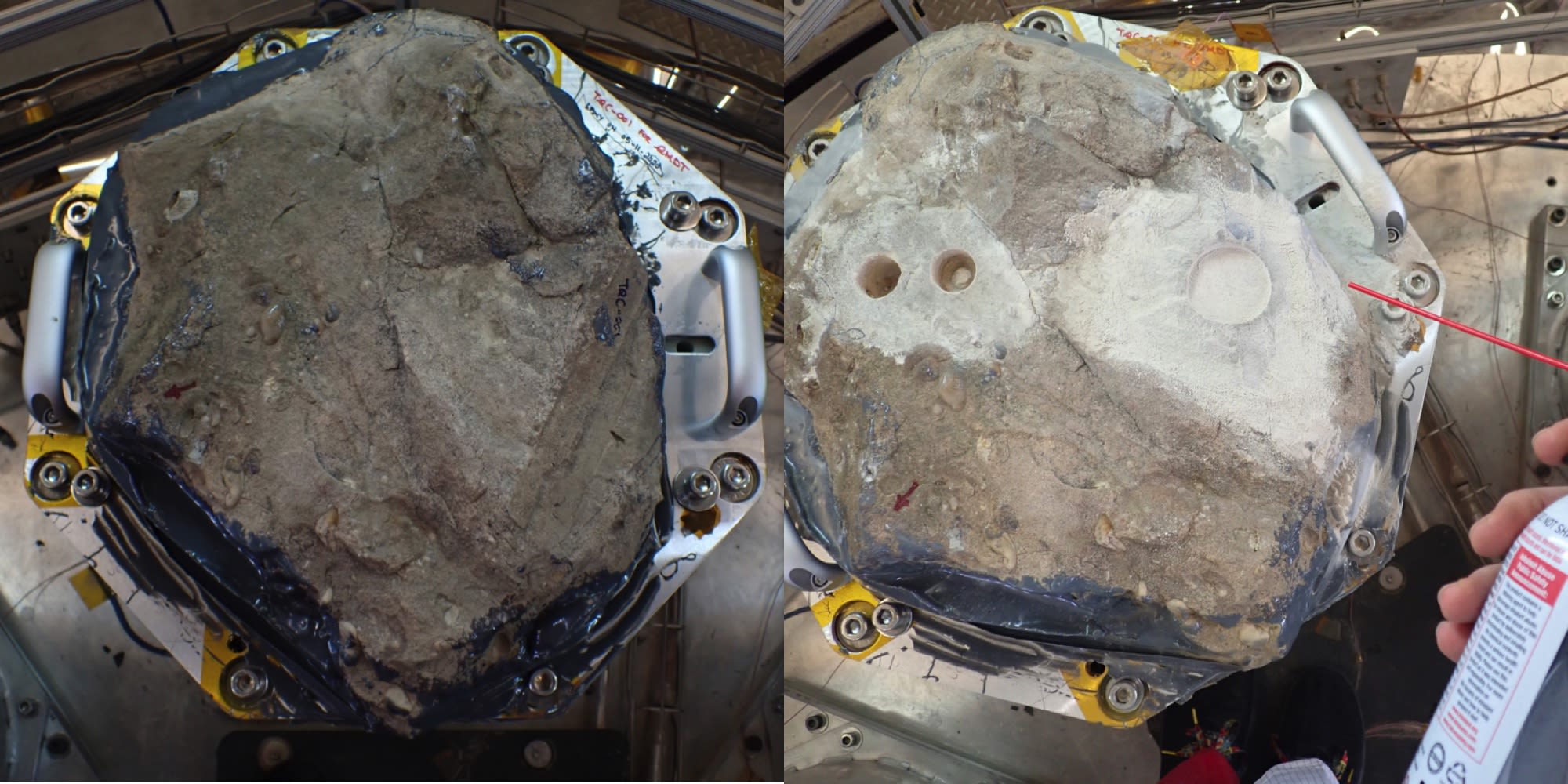
The donated chunk of Sewanee conglomerate before (left) and after coring and abrading in JPL's vacuum chamber. Photos courtesy of JPL.
The donated chunk of Sewanee conglomerate before (left) and after coring and abrading in JPL's vacuum chamber. Photos courtesy of JPL.
On the day we spoke to Williford in late February, he had gotten word that the Sewanee conglomerate had just been abraded and drilled in the latest test session in JPL’s vacuum chamber. Once NASA learns what it can from the rock, it will be shipped back to Sewanee and put on display in Snowden Hall.
For the foreseeable future, Williford will be deeply involved in the planning and operations of the Perseverance rover as it begins its trek across the surface of Mars. Mars days, known as “sols,” are longer than Earth days by about 37 minutes, and the Mars 2020 team operates on a Mars schedule. That means Williford often finds himself meeting with colleagues and evaluating the latest data in the middle of the night on Pacific Time. (During the COVID-19 pandemic, Williford and his family moved from California, where JPL is located, to Seattle, where he’s able to perform all of his responsibilities remotely, including meeting with many of the 450 other Mars 2020 science team members, often for hours a day.)
For all the planning that went into the mission, the team is now adjusting those plans to accommodate facts on the Martian ground. Williford and the rest of the science leadership are working closely with the mission’s rover planners to plot a future course. “We work with a strategic rover planner to evaluate the terrain,” Williford says. “We say we’re here and we want to go there. The rover planner tells us, well, it’s going to take two weeks to get there because you’ve got to go this way around these sand dunes or something. And we say, well that’s going to take too much time, let’s go here instead if that’s easier.”
As new photos and data arrive from Mars daily, Williford cautions his science team colleagues and the public against jumping to any conclusions about what they’re seeing. When Perseverance landed, its cameras showed that just underneath the rover’s wheels were some rocks with holes in them. “One of the first things that people jump to is, ‘Oh, it’s a vesicular basalt! They’re vesicles!’ Which means it’s basalt, which means it’s volcanic. But early on, we decided to call them ‘holey rocks,’” Williford says. “It’s just a really good way to do science anyway, to start with observation and avoid interpretation early on.” So, for now, the most advanced space agency on the planet is categorizing its most recent finds on another planet as “dark rocks,” “light rocks,” and “holey rocks.”
Dark rocks. Light rocks. Hole-y rocks.
— NASA (@NASA) February 22, 2021
Ken Williford, @NASAPersevere deputy project scientist, describes some of the initial observations made by @NASAJPL teams about what the rover has captured from the Martian surface: pic.twitter.com/kcDrQMqS3D
On the day Perseverance landed, Williford was at home in Seattle with his wife and three-year-old daughter, watching the live feed anxiously and waiting for some evidence that the rover made it safely to the surface of Mars. When the first grainy images were transmitted back to Earth and Williford could tell that the wheels were on the ground and the horizon was flat, he and his family leapt to their feet in relief and joy. “When the pictures came up on screen, I was just spontaneously jumping up and down with my daughter and yelling and screaming and crying,” he says. And then, he says, he thought exactly what you might imagine a planetary geologist would think when he realizes he’s about to explore another world: “We’re there! We’re safe! There’s rocks!”
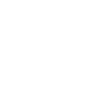